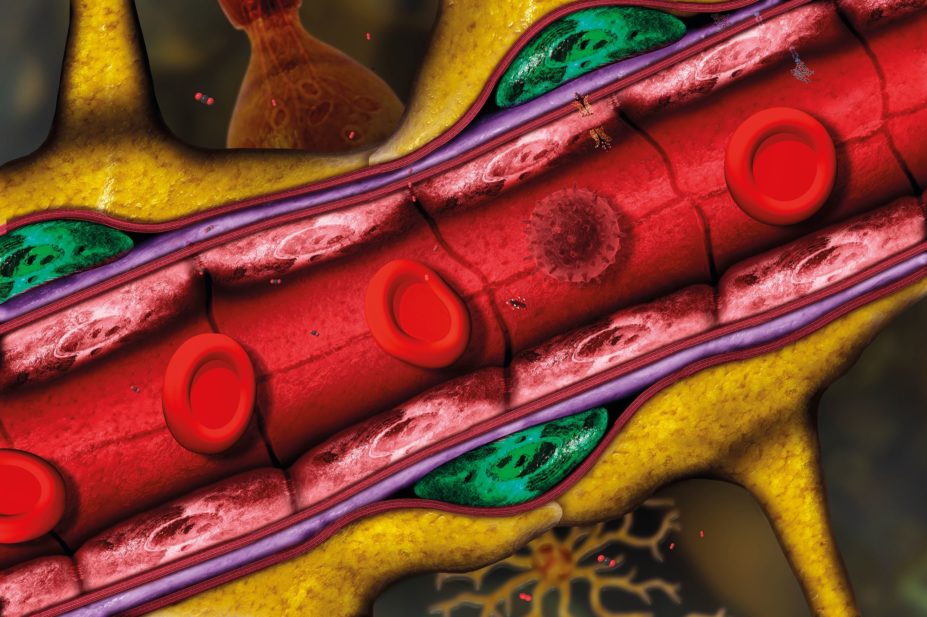
Carol & Mike Werner / Science Photo Library
Medicating the brain is tricky, as pharma giant Eli Lily recently discovered. In 2016, the company abandoned development of its Alzheimer’s drug solanezumab, after it failed several late-stage clinical trials. This adds to a long list of central nervous system (CNS) drug failures. According to a 2014 study, CNS therapeutics take more than a year longer to develop than other drugs and are less than half as likely to obtain marketing approval[1]
. One of the reasons why finding successful neurological therapies is so hard is the blood-brain barrier (BBB) — evolution’s mechanism for protecting the brain.
“The BBB is like a regular cell barrier on steroids,” says medicinal chemist Paul Trippier from Texas Tech University in Lubbock, Texas. It is a lipid membrane that separates circulating blood from fluids in the CNS. Trippier says a good analogy is to think about a child’s ball pit: “If you throw yourself in, you can get to the bottom — that’s like a regular cell membrane. But with the BBB, it’s like throwing yourself against a brick wall.” The wall is made up of closely packed brain endothelial cells, connected by tight junctions that prevent the passage of molecules and ions (see figure 1).
Figure 1: Crossing the blood brain barrier
Source: Pharmaceutics 2014;6:557-583; Nature Reviews Neuroscience 2006;7:41-53
There are several mechanisms by which drugs can cross the blood-brain barrier (BBB):
1. Passive movement of water-soluble agents across the BBB is negligible because of the tight junctions between endothelial cells.
2. Small, lipid-soluble agents, such as antidepressants, cross the BBB via diffusion through endothelial cells.
3. Specialised transport proteins transport glucose, amino acids, and drugs like vinca alkaloids and cyclosporin, across the BBB.
4. Receptors mediate transcytosis of proteins like insulin and transferrin.
5. Proteins, such as albumin, are adsorbed and transported across the BBB by transcytosis.
6. Efflux transporters counter passive diffusion by pumping foreign agents, such as non-sedating antihistamines, out of the brain.
In addition to this, the barrier is policed by a series of bouncers, known as efflux transporters. An example is the transmembrane protein, P-glycoprotein (P-gp), found in intestinal, liver and kidney membranes as well as in the BBB. These proteins kick out foreign molecules that reach the barrier. “That generally is a good thing, because it prevents toxins and other things that we don’t want from getting into the brain,” says Trippier. But these proteins may also remove drug molecules. More recently, the efflux pump BCRP (breast cancer resistance protein), has been found in the BBB. As its name suggests, it was first discovered in breast cancer cells and is responsible for anticancer drug resistance.
The blood-brain barrier is like a regular cell barrier on steroids
Working out how to get drugs through the BBB could be the key to more successful therapies for CNS disorders. Several methods to achieve this are being investigated, from using Trojan horses to smuggle drugs across the barrier to temporarily disrupting the barrier to allow drugs into the brain.
Small molecules
For those familiar with the effects of alcohol and nicotine, it’s clear that some molecules can get through the BBB and medicinal chemists have sought drugs that can also do this. “The classic way goes back to the development of morphine and heroin in the 1890s, and that’s to take small molecules and make them more lipid soluble,” explains physician and researcher William Banks from the University of Washington. “That’s given us 95% of the drugs that we use for the central nervous system.”
Source: Courtesy of William Banks
William Banks, physician and researcher from the University of Washington, says that 95% of the drugs that we use for the central nervous system have been developed by taking small molecules and making them more lipid soluble
More recently, medicinal chemists have taken a more nuanced approach. Size is still important, “most of the clinically available drugs that treat some kind of CNS disease are significantly smaller than other drugs”, says Trippier, whose research focuses on optimising small molecule drugs to pass through the BBB. But charge is another factor, with negatively charged moieties such as acid groups almost impossible to get through. Trippier explains: “If you consider the structure of the lipids that make up the BBB, a negative charge is going to have a lot of interactions and pretty much get stuck on the polar surface.”
For similar reasons minimising hydrogen bonding groups is important, as is reducing the number of rotatable bonds: if you design a floppy molecule that can move around, it has a greater chance of forming hydrogen bonds. Trippier gives the example of the very rigid Alzheimer’s drug memantine (C12 H21 N), which has no rotatable bonds.
In 2010, to help identify compounds with a higher probability of penetrating the BBB, Pfizer chemists came up with the CNS multiparameter optimization algorithm[2]
, which is about 80% predictive, according to Trippier. “If you have an MPO score of four or above, generally it has a pretty good success rate in predicting whether it will get into the brain,” he says. “If the best compounds have three acid groups, we really need to get rid of them, so we see if we can maybe remove one of them, or replace it with something else, and try and increase the MPO score.”
It’s very straightforward to match all these criteria and get some kind of compound into the brain, but we need to have a compound that engages its target and has therapeutic value
However, Trippier cautions that it is important to make sure not to lose drug potency along the way: “It’s very straightforward to match all these criteria and get some kind of compound into the brain, but we need to have a compound that engages its target and has therapeutic value, so we can’t necessarily remove all the offending functional groups.”
Source: Courtesy of Paul Trippier
Medicinal chemist Paul Trippier from Texas Tech University in Lubbock, Texas, is researching how to optimise small molecule drugs to pass through the blood-brain barrier
Hitching a lift: the Trojan horse approach
What about larger molecules — such as the biologics that make up an increasing number of today’s therapeutics? Can they get through the ‘brick wall’? Banks says many natural peptides and molecules such as insulin, transferrin and lipoproteins do pass through via regulated entry mechanisms — a second aspect of the BBB’s function. Regulated entry mechanisms use influx transporter proteins in the barrier. Macromolecules are then transported across cells in several ways, including transcytosis, where molecules are transported in vesicles.
Taking advantage of these mechanisms is the cornerstone of the Trojan horse approach. By attaching a therapeutic molecule to something that is transported across the BBB, the drug cargo can be smuggled through. However, it has not always been successful, says Banks. One of the first attempts was to use the glucose transport system. “People were putting really big molecules on [glucose] and it didn’t work.” He jokes: “It was like having a Volkswagen pull the space shuttle.”
Approaches today often use short amino acid chains called peptides as Trojan horses. In 2007, the first peptide was proven to transport drugs into the brain. The 29-amino-acid peptide RVG29 was derived from rabies virus glycoprotein (RVG) and was able to deliver a gene-silencing RNA (siRNA) molecule to the brain[3]
. Intravenous injection delivered the antiviral siRNA to neuronal cells in mice and, once inside, the payload provided protection against fatal viral encephalitis.
In the past five years, more than 30 BBB ‘shuttle’ proteins have been identified and their efficiency and versatility is increasing. Organic chemist Ernest Giralt, at Barcelona’s Institute for Research in Biomedicine, has put an interesting spin on the approach. He realised that some animal venoms are able to penetrate the CNS and looked at the bee venom peptide, apamin. By removing the chemical groups that block potassium channels, his team created a non-toxic peptide, Mini-Ap4, which was able to transport attached drugs through the BBB[4]
.
Marseille-based biotechnology company Vect-Horus has patents on several peptide Trojan horses, which it calls vectors, and has been working to optimise their ability to deliver drugs. The company started by selecting target receptors in the BBB and screened peptides to find the best matches, explains Jamal Temsamani, director of drug development for Vect-Horus. Proof of principle of its system for transporting a range of molecules has been demonstrated in animal models. Among them is the peptide neurotensin, which can induce therapeutic hypothermia for resuscitation after cardiac arrest or neonatal ischemia, but cannot pass the BBB. Using a peptide-vector, Vect-Horus has designed a conjugate (VH-N439) that is able to get into the brain. The compound is currently in regulatory preclinical studies.
Peptide shuttles seem promising, and pharmaceutical companies are taking notice, for example Vect-Horus has a current collaboration with Sanofi. But as Trippier points out: “The major issue is their lack of generality, so they can take through one particular compound or one particular biologic, but they completely fail on different ones.”
Temsamani concedes: “There is no unique vector that can be used as a universal brain delivery system”.
Nanoparticles
There are other approaches to getting drugs into the brain, such as looking at how particle structure at the nanoscale affects delivery. As with other drug delivery strategies, encapsulating drugs in nanoparticles aims to help deliver the drug to where it’s needed before being degraded.
Nanomerics, a company founded by Ijeoma Uchegbu, a pharmaceutical nanoscientist at University College London, is concentrating on this approach. Its proprietary Molecular Envelope Technology (MET) nanoparticles are engineered from biocompatible polymers. They self assemble into highly stable 50nm nanoparticles that act as a protective molecular envelope for hydrophobic drugs and peptides hidden inside.
The nanoparticles themselves do not cross the BBB, they stick to the capillaries in the brain and then the drug has a longer time to diffuse across the epithelial cells
Source: Courtesy of Ijeoma Uchegbu
Ijeoma Uchegbu, a pharmaceutical nanoscientist at University College London and founder of Nanomerics, says nanoparticles stick to the capillaries in the brain allowing the drug longer to diffuse across the epithelial cells
The nanoparticles have shown potential in treating brain tumours which are currently attacked with very high levels of cytotoxic agents to get enough drug across the BBB. Using MET nanoparticles loaded with the chemotherapy drug Lomustine, Uchegbu was able to increase survival times in mice by about a third, compared with chemotherapy alone[5]
. She says: “The nanoparticles themselves do not cross the BBB, they stick to the capillaries in the brain and then the drug has a longer time to diffuse across the epithelial cells.” A positive charge on the particles helps them stick, but Uchegbu can’t yet explain why the particles seem to stick to the BBB but not other capillaries in the kidney or lungs.
Uchegbu is also using nanostructures to deliver peptides. An example is the peptide dalargin; this synthetic opioid neurotransmitter has morphine-like activity, but is unable to penetrate the BBB. “We derivatised the peptides to add a lipophilic anchor and this caused the peptide to self-assemble into, in this case, elongated fibres 500nm by 20nm,” she explains. “Because their shape is changed, they seem to cross the endothelial barrier to the brain[6]
.” Using amphiphilic derivatives, with both hydrophilic and hydrophobic parts, peptides will self assemble into fibres that are tightly wrapped around a central hydrophobic core. In this form they resist degradation, improving their chances of getting to the brain. Uchegbu says it’s not clear whether the particles cross the BBB as fibres or if they disassemble and are taken up as individual molecules.
Another approach to getting through the BBB is using exosomes, which, according to Banks, is “the hottest area right now and also the newest area”. Exosomes are tiny lipid bilayer-bound bubbles produced in cells — they are the body’s own transport vehicles. This method of transfer has been successfully tried in animal models of Parkinson’s disease, using exosomes extracted from white blood cells. The exosomes were loaded with the enzyme catalase, a potent antioxidant that counters the neuron-killing inflammation responsible for Parkinson’s[7]
. Several companies, are racing to exploit this technology, including an Oxford University spin-out EvOx, which recently raised a £10m investment.
An immune response: using and delivering antibodies
Monoclonal antibodies (mAbs) are ideal drugs because of their specific targeting but they have a hard time getting into the brain. Typically, antibody concentrations are 1,000 times lower in the brain than in the bloodstream. But, Banks says, this isn’t dampening interest within the pharmaceutical industry. “They are enamoured with getting antibodies into the brain,” says Banks. “I can understand that because they have had so much success in treating peripheral diseases with antibodies,” he adds.
In some cases, antibodies can be used as Trojan horses, currently an approach being developed by California biotech company, ArmaGen. Since 2004, the company has developed a pipeline of CNS drugs that fuse a therapeutic protein to an antibody, selected to bind to insulin or transferrin receptors on the BBB. Acting as a shuttle, the antibody therefore enables the fused protein to travel through the BBB. The company has several drugs in clinical trials for treating lysosomal storage disorders.
Also using this approach is Danish pharmaceutical company, Lundbeck. In partnership with Philadelphia-based biotechnology company, Ossianix, it is using shark antibodies as transporters for antibody CNS drugs. “Shark antibodies are useful because they are smaller than human antibodies [a tenth of the size] and you can make them more modular, which means they will be easier to connect together with other antibodies,” explains Kim Andersen, head of research at Lundbeck. In 2017, Lundbeck announced proof of principle in animal studies. “You get a much higher concentration of the therapeutic antibody inside the brain than if we just take the therapeutic antibody without being coupled to the shark antibody,” confirms Andersen.
Source: Courtesy of Lundbeck
Kim Andersen, Lundbeck’s head of research, says the company is investigating the use of shark antibodies as transporters for antibody central nervous system drugs
Another approach is bispecific antibodies — engineered antibodies that can simultaneously bind to two different antigens — one of them being a BBB receptor that transports the whole antibody through the BBB and the other being the therapeutic target. Roche’s biotech subsidiary, Genentech, has created a bispecific antibody that targets the transferrin receptor, to transport the antibody into the brain, and the protein β-secretase 1, an Alzheimer’s target involved in the production of amyloid peptides in the brain. Results in mouse models have been promising[8]
.
Disrupting the BBB
If drugs can’t get through — are there other options? “Probably the third most tried way of getting drugs into the brain is to somehow disrupt the BBB,” says Banks. In fact diseases such as Alzheimer’s and Parkinson’s already compromise the BBB and make it “leaky”, but can scientists find methods to open it up to let in the necessary therapies? In 2016, researchers at Cornell University, New York, discovered that the heart imaging drug Lexiscan (regadenoson) can open the BBB in mice for a brief window, to enable delivery of chemotherapy drugs and antibodies[9]
. It seems to work by interfering with P-gp efflux transporters. The antidepressant drug amitriptyline has also been found to temporarily disrupt the BBB in rats using the same mechanism as Lexiscan[10]
.
The other method of disrupting the BBB is via ultrasound. Several groups are investigating this method, and in 2016 Paris-based company CarThera, published results of a 15-patient clinical trial of its SonoCloud device, which could be commercially available by 2020. The ultrasound implant is surgically inserted into the skull to be activated before chemotherapy. It delivers two minutes of low intensity ultrasound which opens the BBB for six hours and increases drug delivery to the brain from five to seven times.
However, the safety of disrupting the BBB has been questioned. Trippier worries that it could leave the brain vulnerable. “If you take Alzheimer’s as an example, it tends to affect older people on multiple drug regimens to treat multiple issues. So, yes, you could open the BBB, but what about all the rest of the drugs that this person is taking?” he asks. “Equally, if this person has a virus, then opening the BBB could easily let the viral infection into the brain.” Banks agrees: ‘‘It’s unlikely to be a universal solution even if it were to work,” he concurs.
If this person has a virus, then opening the BBB could easily let the viral infection into the brain
There are certainly many approaches being investigated, but Uchegbu says, “we haven’t got a leader at the moment that we can say yes, this is where most of the interesting products are going to come from”.
Temsamani has some frustrations at progress over the past 30 years: “Pharma companies [have] spent billions of dollars on drug development but have failed to deliver effective new brain drugs, despite huge potential markets,” he says.
But Lundbeck’s Andersen is more optimistic. He compares today’s progress in neurological therapeutics to the position of cancer therapies 15–20 years ago.
Banks suggests that the problem lies in a fundamentally wrong, and too narrow, approach to the BBB. There is a misunderstanding about the nature of the barrier, he explains. It is not something to break, but rather something that could be harnessed, through understanding it as a complex dynamic interface with as yet unexplored pathways. ‘If we were to [better] understand how the BBB works and how it transports things that do get into the brain, we could be much further ahead,” he says, adding: “If anyone starts to do this, there could be a renaissance in drug development for the brain.”
References
[1] Tufts CSDD Impact Report. Pace of CNS drug development and FDA approvals lags other classes. November/December 2014. Available at: http://csdd.tufts.edu/reports/impact_reports (accessed May 2017)
[2] Wager TT, Hou X, Verhoest PR et al. Moving beyond rules: the development of a central nervous system multiparameter optimization (CNS MPO) approach to enable alignment of drug-like properties. ACS Chem Neurosci 2010;1:435–449. doi: 10.1021/cn100008c
[3] Kumar P, Wu H, McBride JL et al. Transvascular delivery of small interfering RNA to the central nervous system. Nature 2007;448:39–43. doi: 10.1038/nature05901
[4] Ollerâ€Salvia B, Sánchezâ€Navarro M, Ciudad S et al . MiniApâ€4: a venomâ€inspired peptidomimetic for brain delivery. Angew Chem Int Ed Engl 2016;55:572–575. doi: 10.1002/anie.201508445
[5] Fisusi FA, Siew A, Chooi KW et al. Lomustine nanoparticles enable both bone marrow sparing and high brain drug levels – a strategy for brain cancer treatments. Pharm Res 2016;33:1289–1303. doi: 10.1007/s11095-016-1872-x
[6] Mazza M, Notman R, Anwar J et al. Nanofiber-based delivery of therapeutic peptides to the brain. ACS Nano 2013;7:1016–1026. doi: 10.1021/nn305193d
[7] Haney MJ, Klyachko NL, Zhao Y et al. Exosomes as drug delivery vehicles for Parkinson’s disease therapy. J Control Release 2015;207:18. doi: 10.1016/j.jconrel.2015.03.033
[8] Yu YJ, Atwal JK, Zhang Y et al. Therapeutic bispecific antibodies cross the blood-brain barrier in nonhuman primates. Sci Transl Med 2014;;6:261ra154. doi: 10.1126/scitranslmed.3009835
[9] Kim D-G & Bynoe MS. A2A adenosine receptor modulates drug efflux transporter P-glycoprotein at the blood-brain barrier. J Clin Invest 2016;126:1717–1733. doi: 10.1172/JCI76207
[10] Banks DB, Chan GNY, Evans RA et al. Lysophosphatidic acid and amitriptyline signal through LPA1R to reduce p-glycoprotein transport at the blood-brain-barrier. J Cereb Blood Flow Metab 2017. doi: 10.1177/0271678X17705786